Microbes play a key role in the function of ecosystems. They contribute to biodiversity (Fierer et al. 2012), nutrient cycling (Fenchel et al. 2012), pollutant detoxification (Kolvenbach et al. 2014), and human health (Gevers et al. 2012). Since they control the composition of the gases in the atmosphere, they also play an important role in climate change (Bardgett et al. 2008). In urban ecosystems, microbes account for most of the biodiversity and are major agents in nature’s material cycles and food webs (King 2014). Thus the sustainability of cities over the long term is inextricably linked to microbes and their evolution.
But how does urbanization affect the microbiome? Are urban microbes resilient in the face of rapid environmental changes? This is mostly unknown.
The tiniest urban dwellers can change the planet
Microbes might be the tiniest of urban dwellers, but they are powerful. While known primarily as pathogens and potential threats to human health, microbes play a key role in maintaining major ecological functions that directly support humans and city life. Microbes include bacteria, viruses, archaea, and single-celled eukaryotes such as amoebas, slime molds, and paramecia. Since microbes are invisible to humans, we tend to underestimate their importance in maintaining ecological and human wellbeing.
Microbes play a significant role in the evolution of planet Earth. They have been living on the planet for 3.8 billion years: 2.3 billion years ago, cyanobacteria triggered the Great Oxidation Event, the most significant extinction event in Earth’s history, by producing the oxygen that enabled the evolution of multicellular forms. The extraordinary capacity of microbes to adapt to novel environments makes them particularly interesting to scientists trying to understand evolution on an urbanizing planet. They are tiny and ubiquitous and exhibit vast genetic and metabolic variability as well as great genetic plasticity. These properties allow them to adapt rapidly to unfavorable and changing environmental conditions (Guerrero and Berlanga 2009). Interestingly, microbes rarely act alone; instead, they operate as a team in complex communities (Boon et al. 2013). By building on their metabolic diversity these communities operate as complex networks to perform a variety of ecosystem processes and functions.
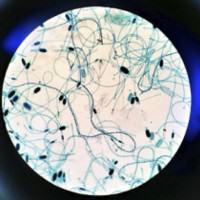
Despite microbes’ remarkable power, we know surprisingly little about them, and even less about those in the urban environment. In fact, scientists are continually discovering new species. Metagenomic sequencing of the urban microbiome will advance our knowledge as we come to understand the genes necessary for survival in urban habitats and learn how their expression enable microbes to adapt to city environments.
The urban microbiome
The urban microbiome is made up of diverse assemblages of resident and transient microbes that inhabit the city (King 2014). Microbes can be found in and on both the natural and built components of urban ecosystems, including the atmosphere, vegetation, open ground, soil, bodies of water, building surfaces, green roofs, indoor environments, and human bodies; microbes inhabit all elements of our city infrastructure including wastewater treatment plants, combined sewer overflow systems, roads, and subway systems (see below).
Scientists are curious to learn whether we can detect a microbial urban signature. We hope to do so by coupling the properties of urban ecosystems with data on microbial community metagenomics. Many empirical studies in urban areas have pointed to the significant role that microbes play in urban soil (Groffman et al. 2002, Kaye et al. 2006, Pouyat et al. 2010), the atmosphere (Brodie et al. 2007, Bowers et al. 2011), and water (Selvakumar and Borst 2006, Jung et al. 2014).
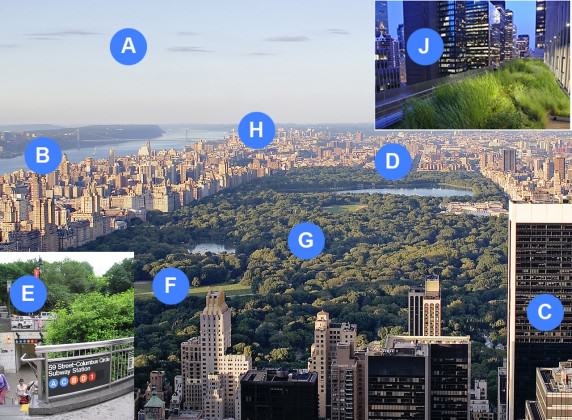
Urbanization affects soil microbes through a variety of abiotic and biotic changes in land use and land cover, and the introduction of variety pollutants. But what influence do different types of land use and human activities have on the properties of soil microbes and on soil quality in urban areas? Some initial findings indicate that the abundance, diversity, and functions of microbes vary across different land uses (Bowers et al. 2011), and their diversity seems to be higher at increased soil depth (Ramirez et al. 2014).
The urban atmosphere harbors highly diverse transient microbial communities. Brodie et al. (2007) examined urban aerosols in two US cities and found at least 1,800 different bacteria. The atmosphere constitutes both a source and a sink for the urban microbiome, and acts as a pathway through which microbes move between urban areas and their surroundings, even between very distant regions (Bowers et al. 2011). Dust storm events in Africa and the Caribbean might move bacteria across oceans (Kellogg and Griffin 2006). Griffin and Kellogg (2004) estimate that ~ 104 bacteria exist per gram of soil and that 1 million tons of airborne soil are moving around the atmosphere each year; that amounts to a total of more than 1016 dustborne bacteria in our atmosphere.
The signatures of airborne microbes might be associated with various types of land use—and the differences in types are likely driven by shifts in the sources of bacteria. Using barcode pyrosequencing, Bowers et al. (2010) determined that the bacteria in the near-surface atmosphere varied significantly across agricultural, suburban, and forest land uses in northern Colorado. In a more recent study Bowers et al. (2011) found that highly diverse bacterial communities were present in the PM2.5 aerosol fraction (fine particulate matter ≤ 2.5μm) from 96 near-surface atmospheric samples collected from cities throughout the American Midwest. The team also found that microbial communities are strongly affected by the season, but that airborne bacteria differ from those in potential source environments such as leaf surfaces and soils. Fecal material, most likely pet feces, is an unexpected source of bacteria in the urban atmosphere.
More and more researchers are documenting the presence of microbes in a diversity of building interiors and external built surfaces (AAAS 2014). In order to examine the mechanisms that shape the indoor microbiome, biologists are teaming up with architects and designers to collect samples in a variety of indoor environments. Most research has focused on environmental factors including humidity and air temperature (Frankel et al. 2012) and the movement of microbes into the built environment from outdoor habitats and organisms, including humans (Hospodsky et al. 2012), pets (Fujimura et al. 2010), and plants (Berg et al. 2014). Others are exploring the role of building design and maintenance. For example, Kembel et al. (2014) found that architectural design might drive the biogeography of indoor bacterial communities.
Central Park microbiome
When Kelly Ramirez and her team started taking soil samples to characterize the soil in Central Park in New York City, they did not expect to find that its microbial diversity was comparable to the biodiversity seen around the world. To characterize the soil microbiome the team collected a total of 596 soil samples, one every 50 meters throughout the 3.4 square kilometers of green space. They analyzed the samples’ pH, moisture content, carbon and nitrogen concentrations and also sequenced 16S and 18S rRNA to characterize the archaeal, bacterial, and eukaryotic composition of the microbiome. Their key finding, published in the Proceedings of the Royal Society (Ramirez et al. 2014), is that urban systems harbor significant amounts of soil biodiversity: Central Park soils contained nearly as many distinct soil microbial phylotypes and types of soil communities as diverse biomes including the Arctic, tropics, and deserts. Also, though the makeup of the microbiome varies widely across the park, they could predict below-ground diversity patterns based on soil characteristics.
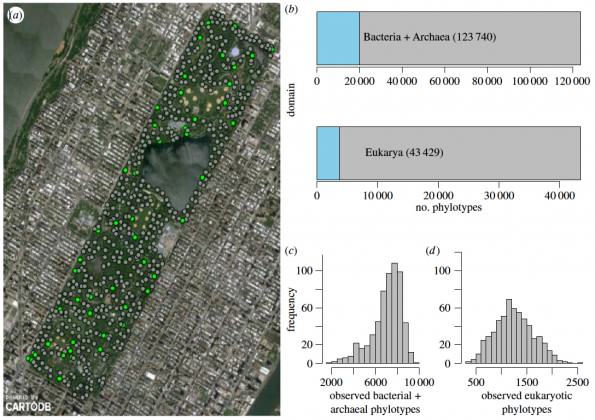
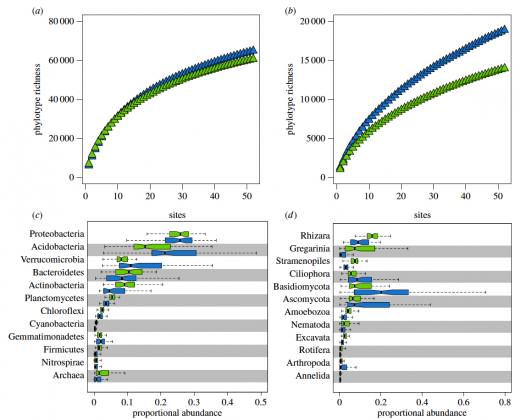
Urban microbes travel on the subway
Recent investigations in Hong Kong and New York City show that urban subways exhibit distinct clusters of microbial communities (Leung et al. 2014). Yet we are only beginning to uncover the mechanisms that shape subway microbiomes across the globe. Microbial diversities within the subway were associated with temperature and relative humidity. The abundance of commuter-associated genera correlated with carbon dioxide levels. The authors also compared the diversity of alpha and beta types and detected different phylogenetic communities associated with different subway lines. The bacterial community within a given subway line could also be correlated with architectural characteristics, outdoor microbiomes, and the degree of connectedness with other lines. Moreover, microbial diversities and assemblages vary within and across days, between peak and non-peak hours.
Ecosystem function
Microbes play key roles in sustaining life on Earth and loss of microbial diversity would significantly affect global and local ecosystems function (Van der Heijden et al. 2007). Although it is well known that microbes drive major biogeochemical cycles and represent the major pool of living biomass in terrestrial ecosystems, ecologists know little about how microbial communities vary across biomes. Scientists are using metagenomic sequencing to unveil some striking patterns. Fierer et al. (2012) examined the functional attributes of samples from 16 soil microbial communities collected from deserts, cold deserts, forests, grasslands, and tundra. They identified the functions the various bacteria performed, including photosynthesis and carbon cycling, and found that the diversity of microbial functions in the soil was directly related to the plant biodiversity above ground.
Microbes have the largest genetic diversity on Earth. Scientists estimate that there are billions of species, although they have described only 1% to 5% of those species. In cities, microbes constitute the greatest pool of genetic biodiversity, providing major ecosystem services that sustain human activities: they treat our wastes, biodegrade a variety of pollutants, and fix nitrogen (King 2014). Thus, loss of microbial diversity could have significant impact on such functions. Soil microbes for example are important regulators of plant community dynamics and plant diversity (Van der Heijden et al. 2007). Although the impact of microbial diversity on plant productivity and diversity is not fully understood, recent studies show that reducing the diversity of soil microbes reduces plant growth. Lau and Lennon (2011) experimentally reduced the complexity of the microbial community in soil, and found that the plants growing there were smaller, had less chlorophyll content, produced fewer flowers, and were less fecund compared to plant populations grown in association with more complex soil microbial communities.
Microbes play also significant roles as agents of both pollution and detoxification. They produce greenhouse gases which contribute to climate change and tropospheric ozone (Bardgett et al. 2008). Yet, since microbial life provides a critical contribution to biogeochemical functions such as decomposition and nutrient cycling (Fenchel et al. 2012), microbes are also a key player in mitigating climate change.
Human Health
Many researchers have established linkages between diseases and the composition of microbiomes (Armougom et al. 2009, Martinez et al. 2013). Recent studies have also found various ways that the human microbiome significantly benefits human health (Ravel et al. 2013). Fierer et al. (2012) points out that the human microbiome constitutes 1014 to 1015 microbial cells. Bacterial cells likely outnumber human cells by at least an order of magnitude, and bacterial genes outnumber the number of genes in the human genome by several orders of magnitude (Qin 2010).
Microbiologists are shifting their attention towards the human microbiome: there are increasing numbers of research projects investigating the relationship between the human microbiome and human health. The National Institute of Health (NIH) Common Fund Human Microbiome Project (HMP) is one of many large scale international initiatives (Methé et al. 2012). Research into the human microbiome and interactions with the urban environment holds significant promise for public health.
Mapping the urban microbiome
Using metagenetic sequencing, scientists have started to map assemblages of microbes that live in urban areas including parks, subways, and buildings, and that live on historical artifacts, cell phones, and other objects, to better understand the urban microbiome (Feazel et al. 2009, Berg et al. 2014, Fujimura et al. 2014, Meadow et al. 2014).
Scientists worldwide use DNA sequence barcodes to catalog the urban microbiome. A project called PathoMap is exploring the microbiome of public spaces in New York City, starting with the subway system. A team at Weill Cornell University in New York City are collecting 1,404 surface samples from 468 NYC subway stations to explore the microbiome at the city level. PathoMap uses a mobile software application from GIS Cloud to record and analyze samples over time. New York University Center for Genomics and Systems Biology and Mount Sinai School of Medicine are collaborating on the study.
The Urban Barcode Research Program (UBRP), led by the Cold Spring Harbor Laboratory, is a science education initiative using barcodes to uniquely identify each species of living thing. It gives high school students an opportunity to study biodiversity using DNA technology. During the 2013–2014 school year, 20 student research teams, representing 17 high schools throughout the five boroughs of New York City, developed projects studying city wildlife, biodiversity, food systems, invasive species, and microbiomes.
How do microbial communities affect urban resilience?
It is well established that the microbiome is important for both the Earth’s ecosystems and human health. Yet we do not know how the stability of the microbiome and its ability to adapt to change relate to the resilience of urban ecosystems. Emerging evidence does indicate that there are symbiotic relationships between microbial communities and human and ecological health, suggesting that microbes play a significant role in maintaining both human and ecosystem functions over the long term. Initial findings show that the urban microbiome is complex, diverse, and dynamic, yet largely unknown at present. Advancing mechanistic understanding of the ecological, evolutionary, and functional implications of anthropogenic impacts on the microbial communities in urban areas pose many challenges and opportunities for preserving ecosystem function and human wellbeing.
A key question is how the urban microbiome influences resilience in urban ecosystems. Although microbial communities are highly adaptable to new conditions, responses to disturbance might shift communities from their initial states to a new state. Understanding the relationship between potential shifts in microbial diversity driven by urbanization and the function and stability of microbial communities will provide important insight towards creating resilience in an urbanizing planet. However, this requires that we expand our knowledge of the diversity and distribution of microorganisms across urbanizing regions.
Recent advances in metagenomics and big data provide new and important opportunities to better understand the invisible life that can influence the future and wellbeing of urban dwellers.
These are some of the questions about urban microbiomes that scientists might focus on, given their new and improved tools:
—How do microbial communities vary across an urban gradient?
—Can we identify an urban microbial signature?
—What mechanisms control these signatures?
—How does the diversity of species affect their function?
—How do the dynamics of microbial communities change with urbanization?
—How can mapping the urban microbiome inform urban planning and design?
Marina Alberti
Seattle
Leave a Reply